Methods Accelerate Biosimilar Analysis
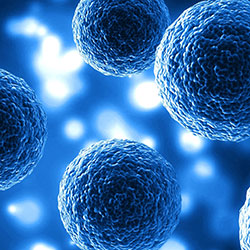
BillionPhotos.com - Stock.Adobe.com
The backbone of biosimilar development is the analytical characterization performed on the innovator—or originator or reference—product, initially to define innovator product attributes and subsequently on the biosimilar in development along with the innovator product in order to demonstrate similarity. In May 2019, FDA published a draft guidance on the analytical assessment and other quality-related considerations for biosimilars, clarifying regulatory requirements and expectations for demonstration of biosimilarity in support of a marketing application (1).
As the foundation in the development of biosimilars is the analytical strategy, this paper reviews effective analytical methodologies available to perform in-depth characterization of both innovator and biosimilar products. Focus is placed mainly on high-resolution mass spectrometry (HR-MS) for primary structural analysis and characterization of post-translational modifications and various biophysical techniques for higher-order structural analysis, while considering the FDA draft guidance.
US biosimilar approval roadblocks
As of Aug. 1, 2019, FDA has approved 21 biosimilars; of these, only five are currently commercially available. Of the approved drugs, five are biosimilars of trastuzumab; three are biosimilars each of infliximab and adalimumab; two are biosimilars each of filgrastim, pegfilgrastim, etanercept, and bevacizumab, respectively; and one is a biosimilar each of rituximab and epoetin.
Currently, the development of biosimilars is lengthy, taking up to seven years to gain approval; the effort is relatively expensive, costing between $150–$200 million per biosimilar product. Identifying analytical methodologies that provide the necessary characterization and product comparability data in a shorter time can reduce the overall product development cycle time and, consequently, development costs. Various analytical approaches can fit the goal of reducing development time and cost for biosimilars.
Mass spectrometry tools
Figure 1. Intact mass profile of innovator product. All Figures Courtesy of the Authors.Mass spectrometry has been a key technology for the characterization of the originators’ products and to perform comparability studies between innovator product and biosimilar molecules. The new generation of mass spectrometers, including time-of-flight (ToF) instruments, as well as hybrid systems such as quadrupole (Q)–ToF, ion trap–ToF or ToF–ToF, and orbital ion trap mass spectrometers offer high mass resolution and mass accuracy.
Using this new generation of equipment introduced in the past five years, it is possible to extract several product attributes by simply determining the intact mass of the molecule. The data provided in Table I and illustrated in Figures 1 and 2 demonstrate product characteristics that can be quickly derived from the determined intact mass profile by a Q-ToF HR-MS.
Figure 2. Intact mass profile of biosimilar product.
From the mass profiles presented in the figures, it can be concluded that both innovator and biosimilar products contain similar proteoforms; of these, the most abundant is the G0F glycoform. While similar species are present in both the innovator product and the biosimilar product, close inspection of peak intensities shows that the innovator product has greater enrichement of the G0F-GlcNAc species relative to the biosimilar. In turn, the biosimilar product displays a higher level of the G2F glycoform relative to the innovator molecule.
Intact mass analysis by HR-MS can provide an abundance of information about the integrity of the molecule and glycoforms comprising the protein product or the presence of oxidation, truncation, or other potential product modification. For more detailed and accurate quantification of these modifications and to identify the sites where these modifications occur, however, it is necessary to perform peptide mapping by liquid chromatography (LC)-MS/MS.
Table I. Summary of proteoforms identified based on intact mass analysis data.
Figure 3. Mirror image of peptide maps of reduced/alkylated and endo-Lys C digested monoclonal antibody product lots (Upper: Innovator product; Lower: Biosimilar product).
Such a quantitative approach, involving large-scale, targeted search of peptide mapping data has been referred previously as a multi-attribute method (MAM) (2). Figure 3 shows the typical output from the peptide mapping of the innovator and biosimilar products of a monoclonal antibody as analyzed with a Q-ToF mass spectrometer. A snippet of the extracted data from the peptide maps is summarized in Table II.
Based on the peptide mapping data, summarized in Table II, the methionine residue at position 256 in the AA253-AA278 peptide is partially oxidized; from the abundance values, it is calculated that 14.9% of this methionine is in the oxidized form. Additionally, the asparigyl residue at position 319 is partially deamidated with approximately 14.1% having converted to the aspartic/isoaspartic form.
Table II. Partial summary of peptide mapping data (innovator product lot).
Furthermore, from the relative measured abundances, it can be quickly determined that, of the glycoforms as summarized in Table II, 97.7% correspond to G0F, 1.3% to G1F, and 1.1% to G2F. It is quite clear from this example that multiple product attributes can be readily extracted and quantified from the intact mass analysis and peptide mapping.
Characterizing higher-order structure
High-resolution tandem mass spectrometry has shown to be quite useful for the characterization of primary structure and both enzymatic and non-enzymatic post translational modifications of biologics. Until recently, higher-order structures, including secondary and tertiary structures, were mostly evaluated by a combination of biophysical techniques, including intrinsic tryptophan fluorescence, as well extrinsic fluorescence, far- and near-UV circular dichroism, Fourier-transform infrared spectroscopy (FTIR), differential scanning calorimetry (DSC), among others. Unfortunately, these methods are low resolution and fail to provide structural details that distinguish among subtle differences in higher-order structures.
It is possible to use multidimensional nuclear magnetic resonance (MD–NMR) or X-ray crystallography to obtain detailed analysis of both secondary, tertiary, and—as applicable—quaternary structures, but these latter methods are very time consuming and quite expensive to run and certainly not suitable to be easily applied for the analysis of multiple lots.
Mass spectrometry has also been found to be quite useful in providing detailed higher-order structural information in a very timely manner. These methods, which include native ion-mobility mass spectrometry (nIM–MS), collision activated ion-mobility mass spectrometry, and hydrogen-deuterium exchange mass spectrometry (HDX–MS), are substantially less time consuming than MD-NMR or X-ray crystallography and can be easily applied across multiple lots for comparability studies. Furthermore, the output from these MS techniques is substantially more informative than the low-resolution biophysical methodologies.
In a publication by Upton, et al., the authors, using the combination of IM–MS, HDX–MS, and collision-activated IM–MS, were able to detect some minute differences in higher- order structures among lots of innovator Herceptin (3). While nIM-MS and, more so, collision-activated IM-MS have gained traction in the past few years in their applicability for analysis of higher structures of biopharmaceutical molecules, HDX–MS has been available for quite some time and has been used in several comparability studies between innovator and biosimilar products (4). With the introduction of more advanced software to analyze HDX–MS data, such as Deuteros (5), it is expected that HDX–MS will become a more widely used tool in the analysis of biosimilars for demonstrating comparability to their respective innovator products.
It is worth noting that IM has also been used for top-down analysis of proteins, even when coupled with medium-resolution mass spectrometers, yielding two-dimensional spectra. By means of two new data analysis programs, IMTBX (IM Toolbox) and Grppr (Grouper), the two-dimensional spectra have been easily and automatically processed to obtain not only full protein sequence but also post-translational modifications (6).
Conclusion
The biosimilar industry in the United States has been evolving more slowly than anticipated and drug competition that would have resulted in lower costs for biologics has yet be realized. One of the reasons for this slow start in biosimilars commercialization is partially due to patent litigation; however, development time and overall costs for biosimilars have certainly played a central role in the failure of biosimilars to meet commercial expectations.
Shortening the development time by using more efficient analytical technologies, as discussed in this paper, not only will speed up the introduction of these follow-on biologics but will also decrease the development costs. Shortening the development cycle and reducing development costs should entice more biopharmaceutical as well as start-up companies to enter the biosimilar space, resulting in increased competition that should translate into reduced costs for these biologics on behalf of the consumers.
References
1. FDA, Draft Guidance to Industry; Development of Therapeutic Protein Biosimilars: Comparative Analytical Assessment and Other Quality-Related Considerations (Rockville, MD, May 2019).
2. R. S Rogers, et al., Mabs, (5), 881-890 (2015).
3. R. Upton, et. al., Chem Sci., 10 (9), 2811-2820 (2019).
4. R.E. Iacob, et. al., LCGC, 35(6), 382-390 (2017).
5. A.M. Lau, et.al., Bioinformatics, 2019, 1-3 (Jan. 14, 2019).
6. D.M. Avtonomov, et. al, Anal. Chem., 2018, 90(3), 2369-2375.